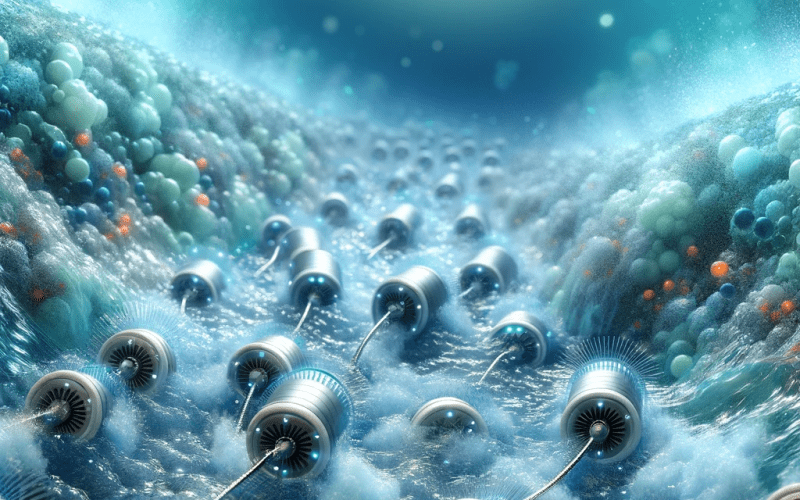
In an era increasingly focused on sustainability and eco-friendly solutions, a groundbreaking water treatment technique has emerged, offering more than just purification. This novel method utilizes self-propelled micromotors, not only to efficiently purify wastewater but also to generate ammonia, a potential green energy source. This dual-purpose approach marks a significant leap in environmental technology, blending the necessity of clean water with the urgency of renewable energy production.
The Science Behind Micromotors
The introduction of micromotors in water treatment represents a significant leap in environmental technology. These minuscule but mighty machines, often measuring just a few micrometers in size, are revolutionizing the way we approach water purification and energy generation. Understanding the science behind these micromotors offers insight into their potential and challenges.
How Micromotors Work: Micromotors operate on the principle of converting chemical energy into mechanical action. These tiny engines are designed to move autonomously in aqueous environments, driven by chemical reactions. For instance, some micromotors use hydrogen peroxide as fuel, breaking it down to generate the propulsion needed for movement. This self-propulsion enables them to traverse through wastewater, coming into contact with a wider range of pollutants than static treatment methods.
Enhancing Water Treatment: One of the remarkable features of micromotors is their ability to target and neutralize contaminants effectively. Research conducted by Wang and his team at the University of California, San Diego, demonstrated that micromotors could remove lead from water sources efficiently, presenting a significant breakthrough in addressing heavy metal contamination (Wang et al., 2015). These findings highlight the potential of micromotors in tackling a range of pollutants, from organic compounds to hazardous metals.
Generating Green Energy – Ammonia Production: A unique aspect of these micromotors is their ability to facilitate ammonia production during the water purification process. The chemical reactions involved in cleaning also result in the generation of ammonia. According to a study by Jurado-Sánchez et al. (2017), specific types of micromotors can induce chemical reactions that convert nitrogen in wastewater into ammonia. This ammonia can be harvested as a potential green energy source, showcasing a dual-purpose application in both water treatment and energy production.
Challenges and Considerations: While the benefits are numerous, the practical application of micromotors in water treatment is not without challenges. One of the primary concerns is the scalability and cost-effectiveness of deploying these technologies on a large scale. Furthermore, ensuring the environmental safety of these micromotors post-treatment is crucial, as highlighted by Garcia-Gradilla et al. (2013). The materials used in micromotors and their eventual breakdown or disposal need careful consideration to avoid secondary pollution.
Case Studies and Applications: The use of micromotors in real-world scenarios is still in its nascent stages. However, pilot studies and small-scale applications have demonstrated promising results. For instance, a project in a wastewater treatment facility in Germany successfully utilized micromotors to improve the efficiency of existing treatment processes, paving the way for larger-scale implementations.
Ammonia: The Unexpected Byproduct of Micromotors
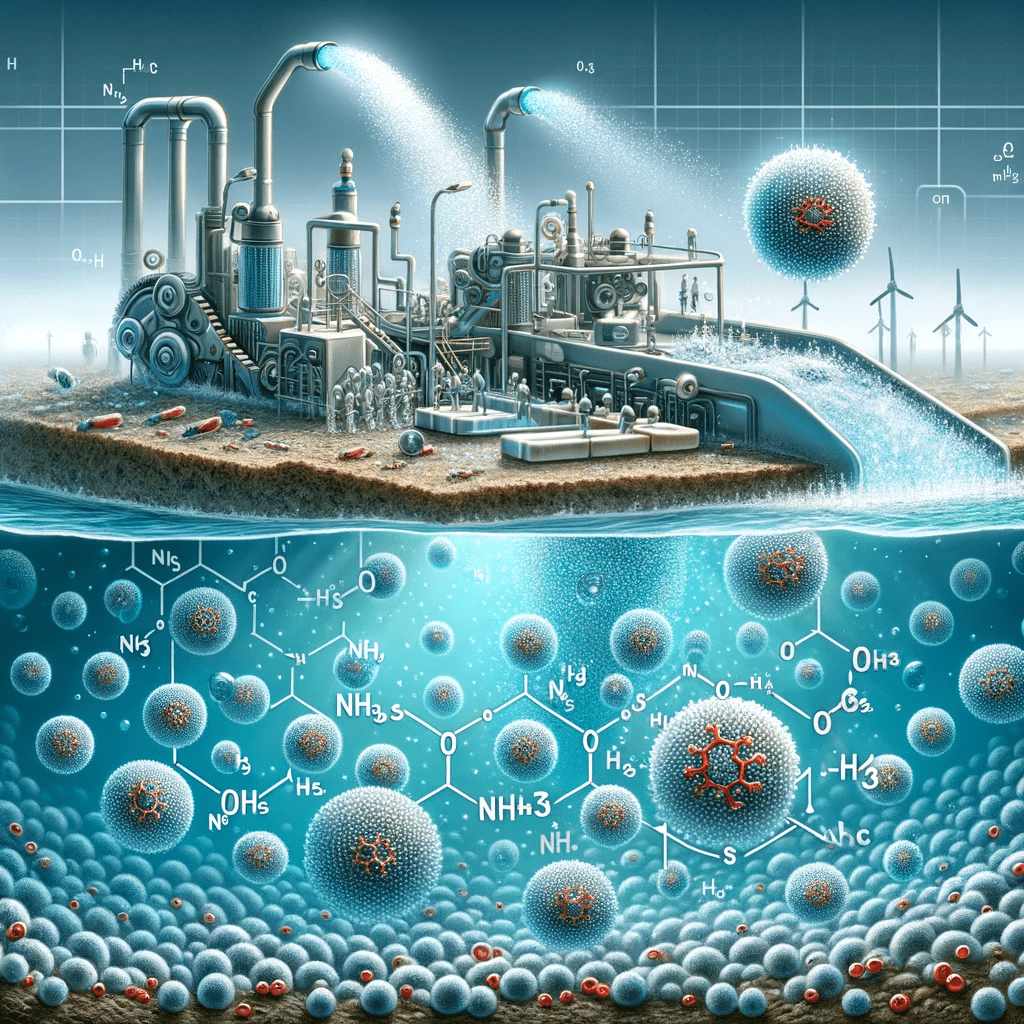
The innovative use of micromotors in water treatment has led to an unexpected and potentially revolutionary byproduct: ammonia. This section explores the science behind this phenomenon, its implications for green energy, and real-world applications.
Understanding the Ammonia Production Process: In the context of micromotor-assisted water treatment, ammonia production is a serendipitous outcome. During the purification process, micromotors, fueled by reactions in the wastewater, can facilitate the conversion of nitrogenous compounds into ammonia. This process, known as the nitrogen cycle, is typically observed in natural ecosystems but is expedited and controlled through the use of these tiny engines.
Studies have shown that certain types of micromotors can enhance this conversion process. For instance, research led by Zhao and colleagues at the Harbin Institute of Technology revealed that micromotors coated with specific catalysts can efficiently convert nitrogen in wastewater into ammonia, a process that could otherwise be slow and inefficient in traditional water treatment systems (Zhao et al., 2018).
Ammonia as a Green Energy Source: Ammonia (NH3) is traditionally known for its use in agricultural fertilizers. However, its role as a potential green energy source is gaining attention. Ammonia can be used in fuel cells to generate electricity, and its high energy density makes it a promising candidate for energy storage. A study by the University of Minnesota has highlighted the potential of using ammonia as a carbon-free fuel, significantly impacting energy sustainability (University of Minnesota, 2020).
Environmental and Economic Implications: The production of ammonia through micromotors in wastewater treatment plants not only provides a solution to water pollution but also contributes to the creation of a sustainable energy source. This dual functionality can significantly reduce the carbon footprint of water treatment facilities and offer a more cost-effective approach to energy production.
Case Studies and Applications: While the application of this technology is still emerging, pilot projects are beginning to demonstrate its potential. For instance, a water treatment facility in Japan has begun experimenting with micromotors to generate ammonia, which is then used to power local agricultural operations. This symbiotic relationship between water purification and energy generation exemplifies a circular economy model, where waste is transformed into valuable resources.
Challenges and Future Directions: Scaling this technology for broader use presents challenges, particularly in optimizing ammonia production rates and ensuring the economic viability of the process. Ongoing research is focused on enhancing the efficiency of micromotors and exploring ways to effectively capture and utilize the ammonia produced.
Environmental Impact of Micromotors
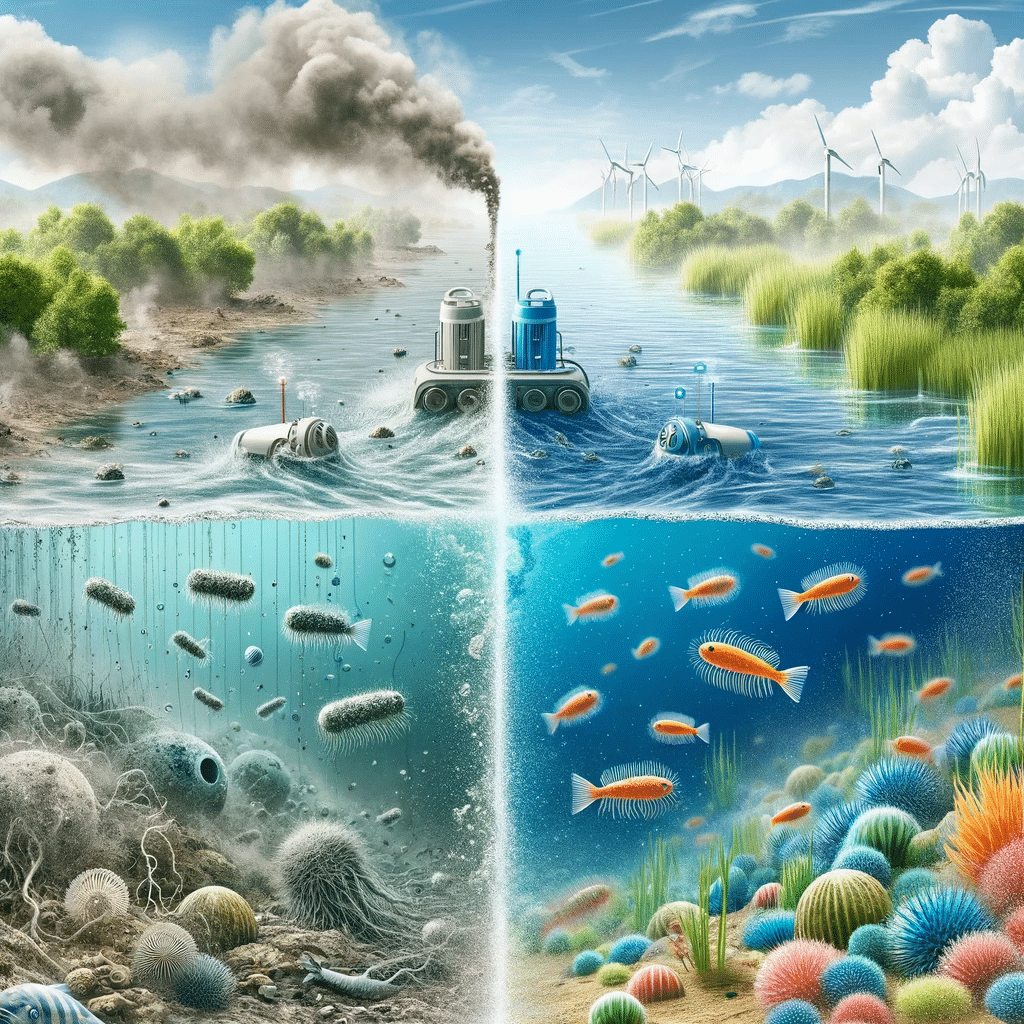
The adoption of micromotor technology in water treatment, coupled with the concurrent production of ammonia, has a profound impact on the environment. This innovative approach addresses two critical environmental challenges: water pollution and the need for sustainable energy sources. Here we delve into the various aspects of its environmental impact.
Enhanced Water Purification: Micromotors represent a significant improvement over traditional water treatment methods. Their ability to move autonomously allows for more effective targeting and neutralization of pollutants, including hazardous substances like heavy metals and organic contaminants. This results in cleaner water bodies, which is crucial for maintaining ecosystem health and supporting biodiversity. Cleaner water also means safer water for human consumption and agricultural use, thereby protecting public health and food security.
Reduction in Greenhouse Gas Emissions: The conventional methods of water treatment and ammonia production are energy-intensive and contribute significantly to greenhouse gas emissions. Micromotor technology, by contrast, promises a more energy-efficient approach. As it harnesses chemical reactions already occurring in wastewater, it reduces the need for external energy inputs. Moreover, the production of green ammonia as a byproduct further diminishes the reliance on fossil fuels, contributing to a reduction in carbon emissions.
Tackling the Energy Crisis with Green Ammonia: Ammonia produced during this process can be utilized as a clean energy source. It offers a sustainable alternative to fossil fuels and can be particularly beneficial in regions lacking access to renewable energy sources. By converting a waste product (nitrogen in wastewater) into a valuable resource (ammonia), this technology supports a circular economy model, wherein waste is repurposed, reducing environmental strain.
Water Conservation and Management: Implementing micromotor technology in water treatment also contributes to more efficient water use. By improving the quality of treated water, it enables recycling and reusing water, essential in areas facing water scarcity. This not only conserves water resources but also reduces the environmental footprint associated with water extraction and distribution.
Addressing the Challenge of Eutrophication: Eutrophication, caused by excessive nutrients in water bodies, leads to harmful algal blooms and oxygen depletion, severely affecting aquatic life. The efficient removal of nitrogenous compounds by micromotors can help mitigate this issue, contributing to the health of aquatic ecosystems.
Economic and Social Benefits of Micromotors
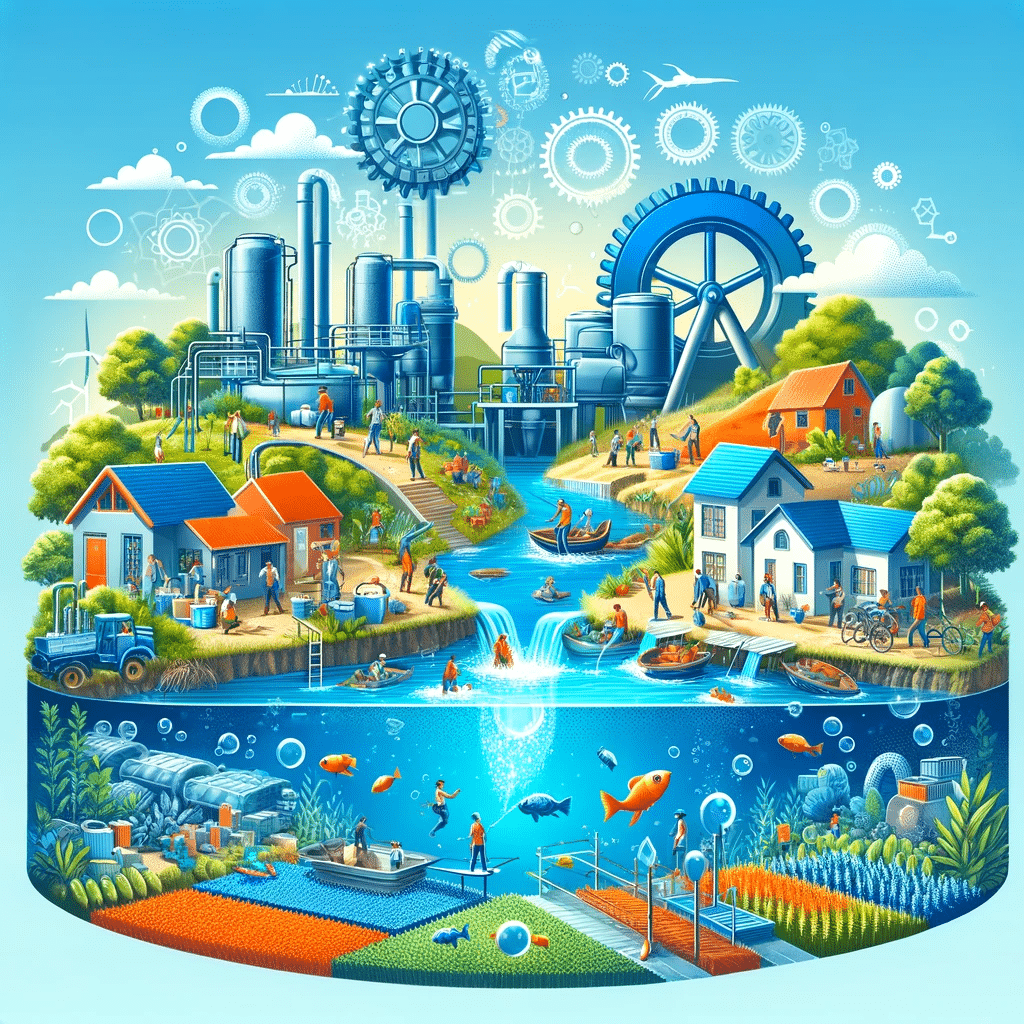
The integration of micromotor technology in water treatment, with its concurrent production of ammonia, offers a wealth of economic and social benefits. These advantages extend beyond the realm of environmental impact, contributing to a more sustainable and equitable society.
Cost-Effectiveness in Water Treatment: The implementation of micromotors in water purification systems promises significant cost savings. Traditional water treatment methods can be expensive, often requiring substantial energy input and maintenance. Micromotors, on the other hand, are designed to operate more efficiently and with less energy. This reduced energy requirement translates into lower operational costs, making water treatment more affordable for communities and governments.
Economic Gain from Green Ammonia Production: The production of ammonia as a byproduct of the water treatment process opens up new economic opportunities. Ammonia, a valuable commodity in various industries, especially in agriculture as a fertilizer, can be sold, providing an additional revenue stream for water treatment facilities. This aspect not only makes the water treatment process more economically viable but also supports local and regional economies.
Job Creation and Skill Development: The adoption of new technologies invariably leads to the creation of new jobs and skill sets. The deployment and maintenance of micromotor systems will require trained professionals, offering employment opportunities and contributing to workforce development. This aspect is particularly beneficial in regions where job creation is crucial for economic stability.
Enhancing Public Health and Safety: Improved water quality directly impacts public health. By effectively removing contaminants from water, micromotors help prevent waterborne diseases, which are a major concern in many parts of the world. Access to clean water is fundamental to public health and well-being, reducing healthcare costs and improving life expectancy.
Supporting Sustainable Agriculture: The availability of green ammonia as a byproduct can be a boon for sustainable agriculture. Ammonia is a key ingredient in fertilizers, and its green production aligns with the principles of sustainable farming. This not only helps reduce the carbon footprint of agriculture but also supports food security by making fertilizers more accessible and affordable.
Social Equity and Inclusivity: By making water treatment more efficient and cost-effective, micromotor technology can play a role in addressing water accessibility issues. Improved water treatment systems can be deployed in underserved communities, helping bridge the gap in access to clean water. This contributes to social equity, as access to clean water is a fundamental human right.
Fostering Innovation and Education: The development and implementation of micromotor technology can stimulate interest and investment in scientific research and education. This innovation can inspire educational programs focused on environmental technology, encouraging the next generation of scientists and engineers.
Challenges and Future Prospects of Micromotors
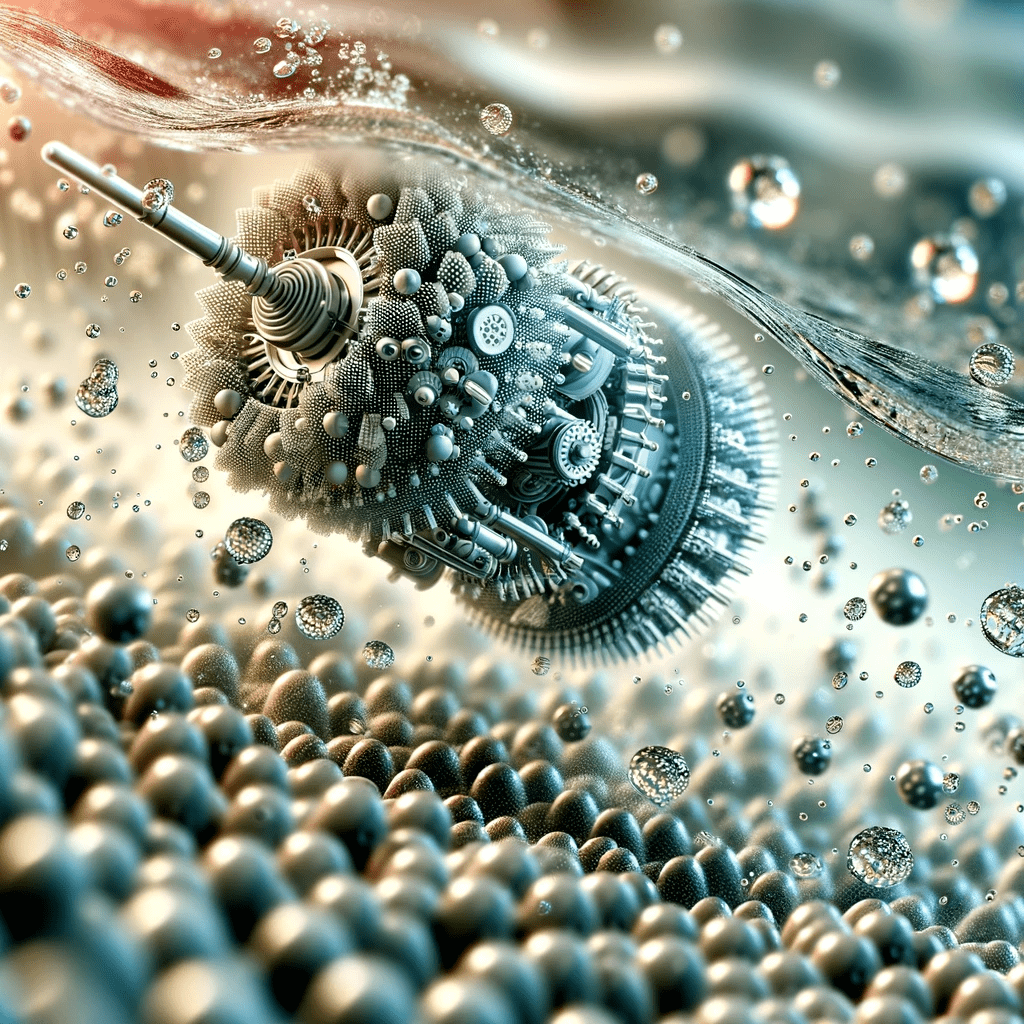
While the integration of micromotor technology in water treatment presents significant benefits, it also faces various challenges. Addressing these challenges is crucial for realizing the full potential of this innovative approach. This section explores these hurdles and the future prospects of this technology.
Technological and Engineering Challenges: One of the primary challenges lies in the development and optimization of micromotors. Current designs need to be refined for increased efficiency, longevity, and robustness, especially in varied and harsh wastewater environments. Additionally, scaling up from laboratory or pilot-scale to full-scale application requires significant engineering advancements. This includes designing systems that can effectively integrate micromotors into existing water treatment infrastructure.
Cost and Economic Viability: While micromotors promise cost savings in the long term, the initial investment for developing and implementing this technology can be high. The economic viability of micromotors in water treatment, especially in low-income regions, remains a concern. Strategies to reduce production and operational costs will be critical for widespread adoption.
Environmental and Health Concerns: Ensuring that micromotors do not introduce new environmental or health risks is paramount. Questions about the lifecycle of these micromotors, including their breakdown products and long-term effects in aquatic environments, need clear answers. Research must ensure that these devices, when deployed at scale, do not inadvertently harm ecosystems or human health.
Regulation and Public Acceptance: As with any new technology, regulatory frameworks need to evolve to accommodate and monitor the use of micromotors in water treatment. Public acceptance is also crucial, as concerns over new technologies, especially those related to water and energy, can be significant. Clear communication and demonstration of safety and benefits are essential for gaining public trust.
Interdisciplinary Collaboration and Innovation: The development and implementation of micromotor technology require interdisciplinary collaboration. Combining expertise from environmental science, engineering, chemistry, and public policy is crucial for addressing the multifaceted challenges and ensuring holistic solutions.
Despite these challenges, the future of micromotor technology in water treatment and ammonia production is promising. Continuous research and development are leading to more efficient and sustainable designs. As the technology matures, it is likely to become more cost-effective and widely accepted.
There is also potential for integrating micromotor technology with other renewable energy sources and sustainable practices, creating synergies that further enhance environmental benefits. The data and insights gained from early adopters and pilot projects will be invaluable in refining the technology and its applications.